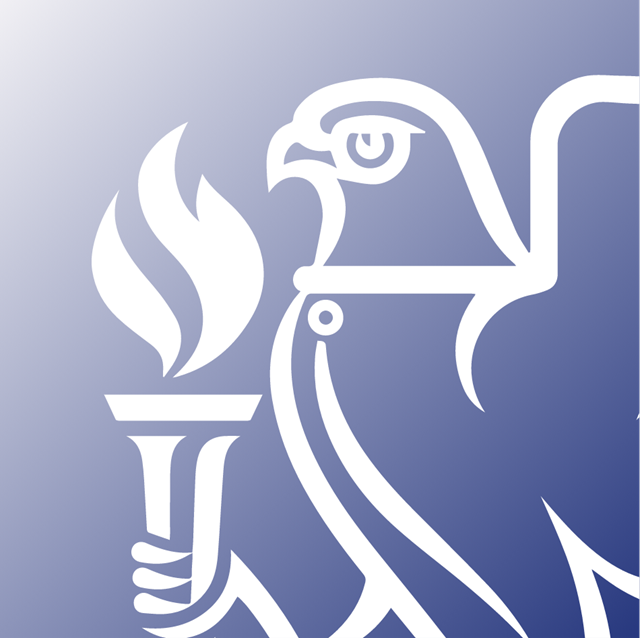
Back to CIBSE Case Studies Overview
Article from the February 2014 edition of the CIBSE Journal written by Craig English.
More than 2,000 athletes at the 2010 Winter Games in Whistler were kept warm by heat extracted from an existing sewage treatment plant. Richard Perry and Tom Ren look at the legacy of their heat network design, and reveal how the project achieved 50% savings in energy use.
The Olympics aren't just about the athletes. For the hosts it is an opportunity to put their cities on the map, and create a string of worldclass new sports venues. New buildings help leverage investment in new infrastructure, giving local authorities the chance to create more effi cient and cost-effective methods of providing energy.
One town to benefit from an Olympic legacy is Whistler in Canada, where a heatrecovery plant was installed to extract energy from a sewage waste treatment plant. The network was used to provide heating and cooling for more than 2,000 athletes and coaches at the 2010 Winter Games. Post-Olympics, it provides energy for hundreds of homes.
The system consists of a central service building, which houses circulating pumps, heat pumps and boilers; a distribution network; heat pumps in each of the residences; and connected heating, cooling and domestic water-heating systems.
Designing the Whistler system was extremely complicated because of the variability of both the energy source and the residential demands. Further complicating matters was the need for the system to perform in a cold climate. The buildings in Phase 1 have been converted for residential use and have been in operation for more than a year, during
which time the connected systems have been monitored on an hourly basis and the results documented.
The primary energy source for heating, cooling and domestic hot water is the district energy sharing system (DESS), which takes low-temperature energy from the existing Whistler Village Sewage Treatment Plant and uses it for the heating and cooling of buildings in the project. The DESS was designed with capacity for a community of 400 residential units and their ancillary services - almost all of which are now sold.
Treated sewage is pumped from the existing treatment plant to an adjacent mechanical plant room, where it is filtered before passing through a bank of heat exchangers. A two-pipe, reversed-return, closed-loop system around the Athlete's Village supplies the energy required for all of the heat pumps in Phase 1 of the project.
Water from the heat exchangers in the mechanical plant room is pumped through high-density polyethylene piping, around the distribution loops, providing the energy source for the heat pumps within each of the village buildings. There are no circulating pumps or control valves between the connected building supply and the return to the DESS, with control governed entirely by the pressure difference between the supply and return mains.
The heat pumps in each unit were selected to provide 60% of the peak capacity for heating and/or cooling, with electric heating elements installed in each building as backup. The largest pipe to discharge from in the system mechanical room is 356mm in diameter.
Phase 1 flow from the mechanical room is maintained at 76 l/s. Flow rate for the completed system will be 101 l/s. Space has been reserved for a future heat exchanger and pump. Two gas-fired standby boilers are located in the mechanical room.
District energy sharing system
The innovative aspects of this project rest in the district energy sharing system (DESS). The system is providing heating, cooling and domestic hot water to a very large development using the energy that is reclaimed from the sewage treatment plant. The first year's operation of the system is producing an energy saving of almost 50%, compared with that of a comparable natural gas system. As the system is expanded and the operation refined, this figure is expected to rise to a 60% saving.
The distribution piping creates thermal storage, which is used by the building heat pumps, functioning in either heating or cooling modes. The use of a non-freezing compound in the system was not considered necessary because of the temperatures maintained in the DESS. It is estimated that the design life of the system will be at least 50 years, and will require minimum maintenance. The first year's operation demonstrated it would be inexpensive to operate.
The system, when completed, is projected to have provided a 70% reduction in greenhouse gases over a comparable district heating system, and up to 3,200MW hours of building energy per year, using energy that would otherwise be wasted.
Air quality and thermal comfort
Indoor air quality and maximum outdoor rate for the housing and hostel was determined using ASHRAE Standard 62.1.2004. Controls were set in each building to provide comfort conditions, according to ASHRAE Standard 55-2007. Each of the attached residential units has its own heat pump or pumps, taking energy from the DESS and distributing it to the individual spaces.
In the summer, when the heat pumps are in their cooling mode, the excess heat in the DESS system is returned to the sewage plant effluent through heat exchangers. The domestic water is heated by the energy drawn from the DESS. Domestic water maintained at 54.4° C is heated by the energy drawn from the DESS. The hostel is somewhat different. The ground floor has been converted into a restaurant, while the upper floors are fitted with bunk beds, which are independently rented. Heat pump recovery units reclaim the heat from all of the exhaust air and use this air as ventilation for the rental areas.
An air-to-water heat pump on the roof provides hot or chilled water for the groundfloor fan coil units. To get the optimal thermal comfort of 60% thermal radiation and 40% thermal convection (ASHRAE HVAC Application 2007), heating is provided by radiant floors. Control set points for temperature are always within acceptable ranges that provide the thermal comfort, according to ASHRAE Standard 55-2004.
Actual results
Phase 1 of the project has completed two full years of operation, with the actual loads experienced being monitored. The connected buildings are 82% residential and 18% commercial/industrial. The loads used in arriving at the savings are actual and, although there have been some hitches caused by start-up, control system adjustment and effluent filtering, they compare favourably with the original calculated figures.
The savings experienced were in part, as a result of the energy extracted from the sewage, the use of variable-speed drives on all of the pumps and the reclamation of energy from the connected buildings. Measurements cover the period from August 2010 to August 2011.
As of June 2013, the DESS has shown a 47% reduction in energy, compared to traditional natural gas heating systems, and a 39% reduction compared to electric systems. The results, based on data received from the installed monitoring equipment, compare initial calculations with total energy savings. The site estimate for the completed project was calculated as being 4.69GW.
This total site load was estimated, using general energy intensity values per floor area and by inserting these figures into inhouse energy software. The daily heating load was initially modelled for the site using 2004 temperature data and assumed balance temperatures. To estimate the total load for the measured year (August 2010 to August 2011), the initial weather data was replaced with 20102011 weather data, and the balance temperature was adjusted such that the modelled DESS loads matched the measured DESS loads.
Cost effectiveness
The capital cost for the completed village's DESS was estimated at US $45m (£2-£3m). A comparable conventional district heating system was estimated at between $25m (£15m) and $33m (£20m) - $20-$25m (£12-15m) for insulated steel pipe and $5-$8m (£35m) for the energy centre. The DESS: uses highdensity polyethylene, uninsulated piping; operates at ambient temperatures; benefits from energy sharing between buildings in heating and cooling modes; and requires fewer capital and operating costs.
Environmental impact
Reduction in greenhouse gases is a key factor in the installation of the DESS. The provision of polyethylene for all of the underground piping and the possible future use of tertiary effluent for nontoxic water distribution - such as treated sewage water used for toilet flushing - are examples of impact reductions. The greenhouse gas (GHG) emissions associated with space heating, space cooling, and domestic hot water were calculated using electricity and natural gas consumption. Electricity in British Columbia is assumed to produce 0.022 tCO2e. The GHG emissions for a 50/50 mix of standard distributed natural gas heating and electric heating and cooling were calculated as 497 tCO2e per annum.
The DESS, using wastewater heat recovery, reduced the typical emissions by 285 tCO2e, which is a 57% reduction, or equivalent to taking approximately 57 cars off the road. On completion, it is projected to provide a 70% reduction in greenhouse gases. CJ
Operation and maintenance
The project is controlled by a technologically advanced ESC automation management system that co-ordinates and optimises the DESS to ensure the maximum of energy saving along with maximum indoor comfort conditions.
The control system utilises a fully open network protocol (BACNET), communicating with multiple distributed control panels, including thirdparty manufacturers controls (supplied with the units), for a fully integrated seamless control system. The DDC control system is monitored by the wastewater plant operators and independently by an outside consulting firm.